Understanding Quantum Computing: The Future of Information Processing
Willow
DIGITAL PLATFORM
12/12/20248 min read
Introduction to Quantum Computing
Quantum computing represents a significant departure from traditional classical computing methods. At its core, quantum computing harnesses the principles of quantum mechanics, which govern the behavior of matter and energy at atomic and subatomic levels. Unlike classical computers, which process information in binary, using bits that represent either 0 or 1, quantum computers employ quantum bits, commonly referred to as qubits. This innovative utilization of qubits enables quantum computers to perform complex calculations at speeds unattainable by their classical counterparts.
The fundamental distinction between classical and quantum computing lies in the concept of superposition. While classical bits can only exist in one state at a time, qubits possess the unique ability to exist simultaneously in multiple states, thanks to superposition. This property allows qubits to process a vast amount of information concurrently, dramatically enhancing computational power. Consequently, quantum computers can execute algorithms that solve complex problems, such as factoring large numbers or simulating molecular structures, more efficiently than classical systems.
Another crucial aspect of quantum computing is entanglement, a phenomenon where qubits become interlinked, resulting in a state where the information of one qubit is dependent on the state of another, regardless of distance. This interconnectedness can lead to exponential increases in processing power and has implications for secure communication systems. The combination of superposition and entanglement positions quantum computing as a revolutionary technology, with potential applications across various fields, including cryptography, material science, and optimization problems.
Overall, quantum computing signifies a paradigm shift in information processing. By leveraging the principles of quantum mechanics, it opens new frontiers for computation, leading to advancements that were previously thought unattainable. Understanding these foundational aspects of quantum computing sets the stage for exploring its practical applications and implications in the future.
The Basics of Qubits and Superposition
At the heart of quantum computing lies the concept of qubits, which are the fundamental units of quantum information. Unlike classical bits that can exist in one of two states—0 or 1—qubits can exist in a state of superposition, allowing them to represent both 0 and 1 simultaneously. This unique property arises from the principles of quantum mechanics, enabling a remarkable increase in computational power and efficiency. In a classical system, a bit can only perform one operation at a time, but a qubit can perform multiple calculations at once, fundamentally changing the landscape of information processing.
Superposition is likened to spinning a coin; while it is in motion, it can be considered both heads and tails until it settles into a definitive state upon measurement. This metaphor illustrates the potential of qubits, as they can exist in a combination of states, thereby expanding the overall computational capacity of quantum systems. The mathematical representation of a qubit is achieved using a linear combination of the basis states, symbolically denoted as |0⟩ and |1⟩. This enhancement permits quantum computers to explore a vast solution space, rendering them exceptionally powerful for specific types of problems.
The implications of superposition for quantum computing are profound. For instance, in applications such as cryptography, optimization problems, and complex simulations, the capability to analyze multiple outcomes simultaneously provides a significant advantage over classical computing approaches. As researchers develop quantum algorithms, they harness the concept of superposition to perform operations that would take classical computers an impractical amount of time to solve. Consequently, the use of qubits in a state of superposition not only redefines the way we understand computation but also heralds a new era in technological advancement.
The Exponential Speed-Up of Quantum Computing
Quantum computing represents a significant shift in the realm of information processing, offering computational advantages that classical computers cannot match. The primary distinction lies in quantum computers' ability to exploit the principles of quantum mechanics, particularly superposition and entanglement. This allows quantum machines to perform multiple calculations simultaneously, a feature that underpins their potential for exponential speed-up in solving specific types of problems.
In classical computing, data is processed in binary systems, using bits that can either be in a state of 0 or 1. Quantum computing, on the other hand, utilizes quantum bits, or qubits, which can exist in multiple states at once due to superposition. As a result, a quantum computer with just a few qubits can represent an immense amount of data simultaneously, vastly improving computational efficiency. This leads to faster processing times for complex algorithms, particularly in fields such as cryptography, optimization, and drug discovery.
Another crucial aspect of quantum computing is entanglement, a phenomenon where the state of one qubit becomes linked to the state of another, regardless of the distance separating them. This property enables quantum computers to perform intricate calculations that would take classical computers an impractical amount of time. For instance, certain algorithms, such as Shor’s algorithm for factoring large numbers, showcase how quantum systems can outperform classical counterparts by several orders of magnitude when properly harnessed.
However, while quantum computing holds tremendous promise, it is essential to recognize that it is not universally superior to classical computing. Some problems can still be solved more efficiently by classical systems. Nevertheless, for specific tasks, the exponential speed-up potential of quantum computers indicates a transformative future in computational capabilities and applications across numerous disciplines.
Applications of Quantum Computing
Quantum computing holds the potential to revolutionize various industries, leveraging its unique properties to tackle complex problems that classical computing struggles to solve. Among its most promising applications is drug discovery, where quantum algorithms can simulate molecular interactions at an unprecedented scale. This capability can significantly accelerate the identification and optimization of new drugs, ultimately leading to more effective treatments and lower development costs.
In the realm of materials science, quantum computing enables researchers to model and analyze materials at the atomic level. This can provide insights into new materials that possess desired properties, such as superconductors or advanced alloys. By employing quantum simulations, scientists can predict how materials will behave under different conditions, thereby expediting the development of innovative solutions for industries ranging from aerospace to electronics.
Artificial intelligence (AI) also stands to gain considerably from advancements in quantum computing. Quantum algorithms can enhance machine learning processes, improving the ability of AI systems to analyze vast datasets. This enhancement could lead to more accurate predictions in fields like finance, healthcare, and marketing. For instance, quantum-enhanced AI could optimize supply chain management by analyzing complex variables in real time, providing businesses with competitive advantages.
Furthermore, quantum computing offers significant improvements to cryptography. Traditional encryption methods are increasingly vulnerable to the advanced computational power of quantum systems. By developing quantum encryption techniques, organizations can ensure data security against future threats. Techniques such as Quantum Key Distribution (QKD) utilize the principles of quantum mechanics to create secure communication channels that are practically impervious to interception.
These diverse applications illustrate the transformative potential of quantum computing across various sectors, addressing challenges that were previously beyond reach. As research in this field continues to advance, industries can expect to harness this technology for substantial benefits, paving the way for innovations that will shape the future.
Challenges and Limitations of Quantum Computing
Quantum computing holds significant potential for revolutionizing information processing, yet it also faces numerous challenges and limitations that must be addressed to realize its full capabilities. One of the foremost issues concerns error rates inherent in quantum computations. Quantum bits, or qubits, are susceptible to interference and decoherence, leading to errors in calculations. Unlike classical bits, which remain stable, qubits can exist in multiple states simultaneously, making them more vulnerable to environmental disturbances. As a result, developing error correction methods that can handle these fluctuations is crucial for reliable quantum computing.
Another critical challenge is maintaining qubit coherence. Coherence refers to the ability of qubits to retain their quantum state long enough to perform calculations. Currently, many quantum systems experience rapid loss of coherence, which limits the depth and complexity of computations that can be achieved. Ongoing research is focused on enhancing coherence times, including exploring new materials and techniques to isolate qubits from their environments, thereby prolonging their operational stability.
Moreover, there is an urgent need for robust quantum algorithms that can effectively utilize the unique capabilities of quantum computing. While some algorithms, such as Shor's algorithm for factoring and Grover's algorithm for searching unsorted databases, demonstrate significant advantages over classical counterparts, many problems remain where effective quantum solutions are still under development. Researchers continue to investigate various algorithmic frameworks to fully harness the power of quantum systems.
Efforts are underway across the global scientific community to address these challenges. Collaborative initiatives are seeing physicists and computer scientists work together to create more reliable systems, reduce error rates, improve qubit coherence, and develop practical algorithms. By fostering innovation in these areas, the field of quantum computing aims to lay down a strong foundation for future breakthroughs in information processing technology.
The Future of Quantum Computing
Quantum computing is emerging as a transformative technology with the potential to revolutionize various domains including cryptography, materials science, and artificial intelligence. As we look to the future, several predictions about its evolution can be made. First, advancements in quantum hardware are expected to lead to the development of more stable and scalable quantum processors. Researchers are actively working on overcoming current challenges, such as error rates and coherence times, which will result in quantum computers capable of performing more complex calculations.
In parallel, the algorithmic landscape is poised for growth. The emergence of quantum algorithms that efficiently solve problems intractable for classical computers will likely drive interest and investment in quantum computing. Companies and academic institutions are focusing on developing specialized algorithms that can leverage quantum principles, such as superposition and entanglement, to provide unprecedented speed in processing vast amounts of data. This can have groundbreaking implications for sectors that depend on data, such as finance and pharmaceuticals.
Furthermore, we can anticipate a gradual integration of quantum computing with existing technologies. As quantum systems become more accessible, the convergence with classical computing will likely facilitate hybrid models that utilize both paradigms for optimal performance. The potential for developing quantum cloud services will make these powerful systems available to broader audiences, including small businesses and research organizations, thus democratizing access to quantum resources.
Lastly, the ethical and regulatory frameworks surrounding quantum computing will evolve as the technology matures. Policymakers and technologists will need to collaborate to address concerns about security and ethical implications, ensuring that this revolutionary technology is deployed responsibly. In conclusion, the future of quantum computing holds immense promise, with advancements poised to impact numerous sectors and redefine the landscape of information processing.
Conclusion
Quantum computing represents a significant leap forward in information processing technology, poised to revolutionize various fields by harnessing the principles of quantum mechanics. Throughout this blog post, we have explored the fundamental concepts that underpin quantum computing, including superposition, entanglement, and quantum gates. These principles differentiate quantum processors from classical computers, offering unparalleled speeds and the capacity to solve complex problems that are currently intractable.
Furthermore, we have examined the potential applications of quantum computing across diverse sectors such as cryptography, drug discovery, artificial intelligence, and optimization problems. The transformative potential of quantum technology underscores the necessity for continued research and development, as these advancements promise to drive significant innovations and efficiencies in future applications. As researchers, academia, and industries worldwide intensify their focus on quantum technologies, the rapid evolution of this field invites speculation about the implications it holds for our society and economy.
It is vital for stakeholders, including researchers, policymakers, and the general public, to remain informed and engaged with the developments in quantum computing. Collaboration among scientists and technologists is crucial to overcoming challenges, such as error correction and scalability, that currently limit the practical deployment of quantum computers. As we stand at a pivotal moment in technological history, fostering an understanding of quantum computing and its corresponding advancements can empower us to shape its future positively.
In essence, the journey of quantum computing is just beginning, and its evolution will significantly influence various aspects of our lives. Keeping abreast of research breakthroughs and market developments will be essential for those wishing to understand the future landscape of technology and information processing.
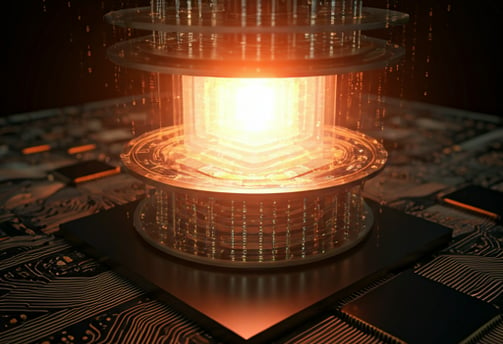
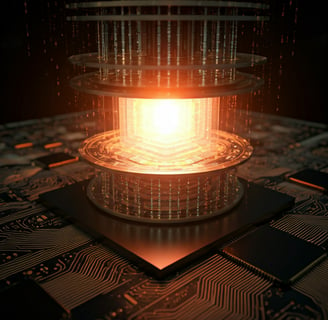